In the article “A Review on the Role of Metabotropic Glutamate Receptors in Neuroplasticity Following Psychostimulant Use Disorder” by Roghayeh Mozafari et al., the authors look at the neurobiological mechanisms behind addiction. They specifically focus on metabotropic glutamate receptors (mGluRs) and their role in neuroplasticity. The review talks about the changes in brain structure and function in response to psychostimulant use. This suggests that mGluRs (which regulate synaptic plasticity) could be a target for treating addiction. The research mentions that drug use can affect the brain’s ability to adapt and heal, which brings forth the need for new approaches to treating addiction. These new ideas would need to be based on the neural pathways that are altered by repeated drug use [1]. In this blog post, we will look at the similarities and differences of addiction to both legal substances (sugar) and illicit (hard drugs).
Addiction Beyond Hard Drugs
Addiction is an increasingly common issue, but not all addictions are created equal. Most people think of addiction in the context of hard drugs (like cocaine, heroin, and methamphetamine), but there’s another type of addiction that is often under the radar: sugar addiction. The question is- is just as dangerous or damaging as addiction to harder substances? How do these addictions compare on a biological level? What do the latest findings in addiction research say about the brain’s response to substances, both legal and illicit?
Why Are These Addictions So Hard to Break?
Addiction to both sugar and psychostimulants alters the brain’s reward system, which causes changes in behavior and cognition. Research has shown that chronic use of both sugar and hard drugs can result in similar neuroplastic changes [2]. These are alterations in the brain’s neural pathways that make it harder for the individual to resist the substance. This neuroplasticity is regulated by metabotropic glutamate receptors (mGluRs), which are involved in both learning and memory [1]. The review by Mozafari et al. shows how these receptors could play a large role in addiction and neuroplasticity by mediating the long-term changes in brain function following repeated psychostimulant use.
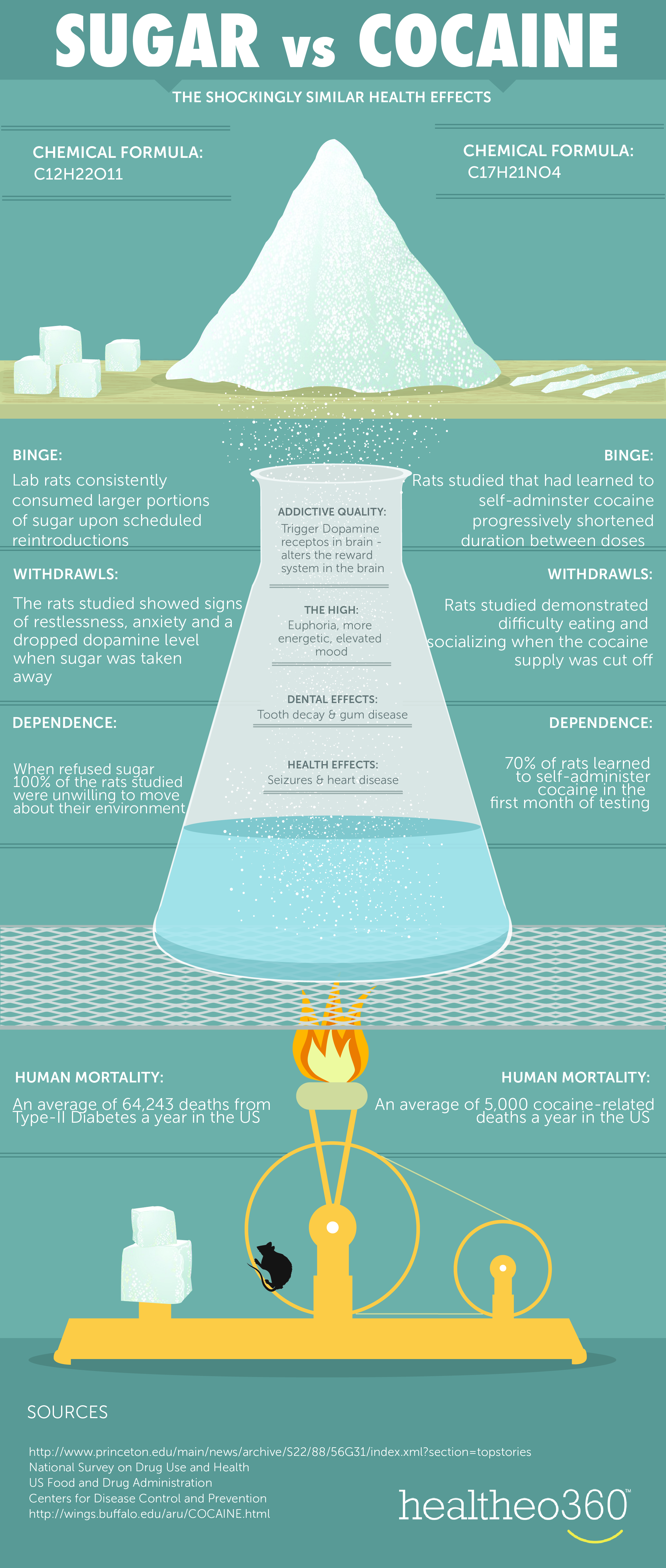
Sugar and Hard Drugs
What Mozafari and colleagues’ findings about addiction in a general sense show is the similarity between sugar addiction and addiction to psychostimulants on a neurological level. Both types of addiction appear to use the same brain systems, while both have neuroplastic changes leading to the development of compulsive behaviors [1]. This suggests that our understanding of addiction should not be limited to illegal drugs but must also include substances like sugar that can trigger similar neural pathways and behavioral outcomes.
What does this mean for future research? Firstly, it opens up new opportunities for studying addiction in a broader context. If sugar addiction involves the same receptors and pathways as hard drugs, then treatments designed for one may be applicable to the other. This could lead to newer therapies for individuals struggling with sugar addiction, such as patients with obesity or type 2 diabetes.
What Does This Mean for You?
The implications of these findings are far-reaching. Beyond the lab and clinic, this research challenges how we think about addiction and its treatment in everyday-life. If addiction to sugar works similarly to hard drugs in terms of its impact on the brain, then it’s very important to reconsider how we approach nutrition and public health. This could spark conversations about food regulations and marketing practices, especially when it comes to dangerously sugary foods and beverages that are normalized in our culture.
It’s beyond time to think about addiction recovery in a more holistic way. Perhaps we need to expand our focus beyond just illicit substances and start viewing sugar as a more widespread and dangerous addiction- one that has long-term impacts for health and society.

Rethinking Our Approach to Addiction
The main takeaway is clear: addiction is not confined to illegal drugs alone. Substances like sugar can hijack the brain in similar ways. This leads to lasting changes in behavior and cognition similarly to hard drugs. The research into metabotropic glutamate receptors offers a new way to view addiction, which suggests new possibilities for treatments.
So, what’s next? It’s important to think critically about our habits, diet, and the systems that support addictive behaviors. The challenge is real, but so is the opportunity to understand and fight addiction in new ways. Stay curious and keep pushing the boundaries of what we know to help create healthier, more informed lives.
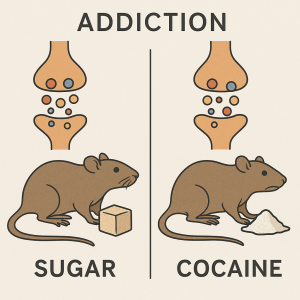
[1] Mozafari, R., Karimi-Haghighi, S., Fattahi, M., Kalivas, P., & Haghparast, A. (2023). A review on the role of metabotropic glutamate receptors in neuroplasticity following psychostimulant use disorder. Progress in Neuropsychopharmacology & Biological Psychiatry, 124. https://doi.org/10.1016/j.pnpbp.2023.110735
[2] Avena NM, Rada P, Hoebel BG. Evidence for sugar addiction: behavioral and neurochemical effects of intermittent, excessive sugar intake. Neurosci Biobehav Rev. 2008;32(1):20-39. doi: 10.1016/j.neubiorev.2007.04.019. Epub 2007 May 18. PMID: 17617461; PMCID: PMC2235907.